Quantum physics is a strange yet intriguing field where particles exist in multiple states simultaneously. You'll encounter concepts like wave-particle duality, which shows that particles can behave as both waves and chunks of matter. Then there's quantum entanglement, where particles instantly affect each other's states, regardless of distance. The Heisenberg Uncertainty Principle reveals that you can't know a particle's exact position and momentum at the same time. This challenging yet fascinating world transforms our understanding of reality, inviting you to explore its mysterious depths and uncover how these principles impact our technology and daily lives.
Key Takeaways
- Quantum physics studies matter and energy at atomic levels, revealing phenomena like wave-particle duality and quantum entanglement.
- Superposition allows particles to exist in multiple states until observed, leading to unpredictability in measurement outcomes.
- Quantum entanglement demonstrates how particles can instantaneously affect each other's states over vast distances.
- The Heisenberg Uncertainty Principle highlights limits on measuring a particle's position and momentum simultaneously, emphasizing quantum probabilistic nature.
- Practical applications of quantum physics include lasers, MRI scanners, and quantum computing, driving technological advancements.
What Is Quantum Physics?
Quantum physics is the fascinating branch of science that dives into the behavior of matter and energy on the tiniest scales, like atoms and subatomic particles.
You'll discover that quantum mechanics reveals a world unlike anything you experience in everyday life. For instance, particles such as photons exhibit wave-particle duality, acting like both waves and particles depending on how you observe them. This bizarre behavior challenges our classical understanding of physics.
Additionally, the principles of quantum physics have significant implications in various fields, including AI in Cybersecurity, where advancements in technology are driven by quantum algorithms.
One key concept you'll encounter is the Heisenberg uncertainty principle, which states that you can't precisely know both the position and momentum of a particle at the same time. This limitation reshapes how we think about measurement in the quantum domain.
Moreover, quantum entanglement showcases the interconnectedness of particles. When two particles become entangled, measuring one instantly affects the state of the other, no matter how far apart they are. This phenomenon defies classical intuition and hints at deeper connections in the universe.
In essence, quantum physics not only reshapes scientific understanding but also influences modern technology through innovations like lasers and quantum computing.
It's a strange and exciting field that continues to challenge and inspire.
Key Principles of Quantum Mechanics
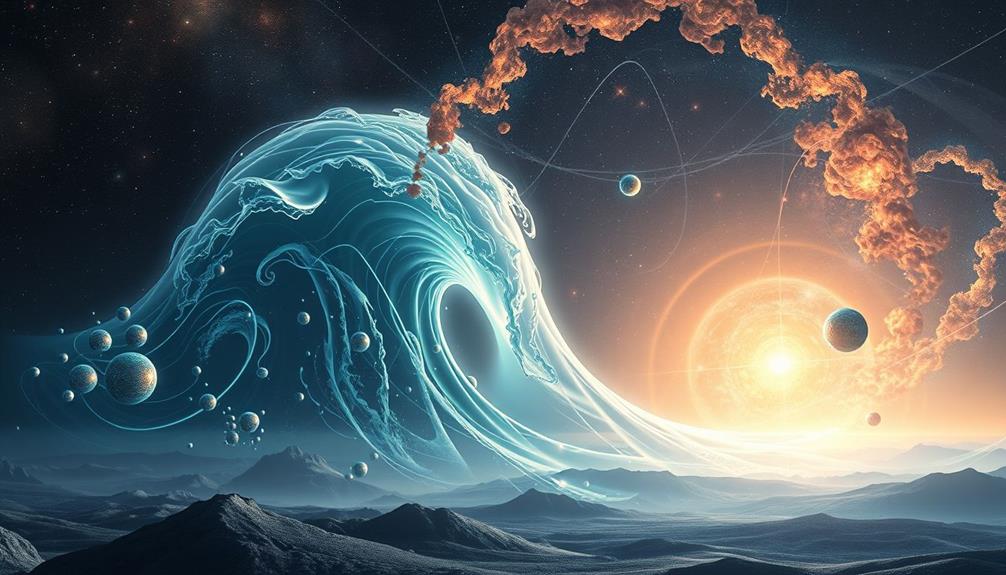
Plunge into the key principles of quantum mechanics, where you'll encounter some of the most mind-bending concepts in physics. One of the foundational ideas is superposition. This principle allows a quantum state to exist in multiple states simultaneously, as famously illustrated by Schrödinger's cat thought experiment.
Until observed, the cat is both alive and dead, showcasing the strange reality of quantum behavior. Curiously, this concept shares parallels with phenomena such as sonoluminescence secrets, where bubbles in a liquid emit light through energy fluctuations.
Another essential concept is wave-particle duality, where particles like photons exhibit both wave-like and particle-like properties. This duality can be witnessed in the double-slit experiment, revealing the complexities of their nature.
Then there's quantum entanglement, a phenomenon that connects particles in such a way that the state of one instantly influences the state of another, no matter the distance separating them. This interconnectedness defies our classical understanding of locality.
Lastly, the Heisenberg uncertainty principle emphasizes the probabilistic nature of quantum states, asserting that you can't precisely know both the position and momentum of a quantum object at the same time.
These principles collectively challenge our traditional perceptions and invite you to rethink the very fabric of reality.
Understanding Quantum Measurement
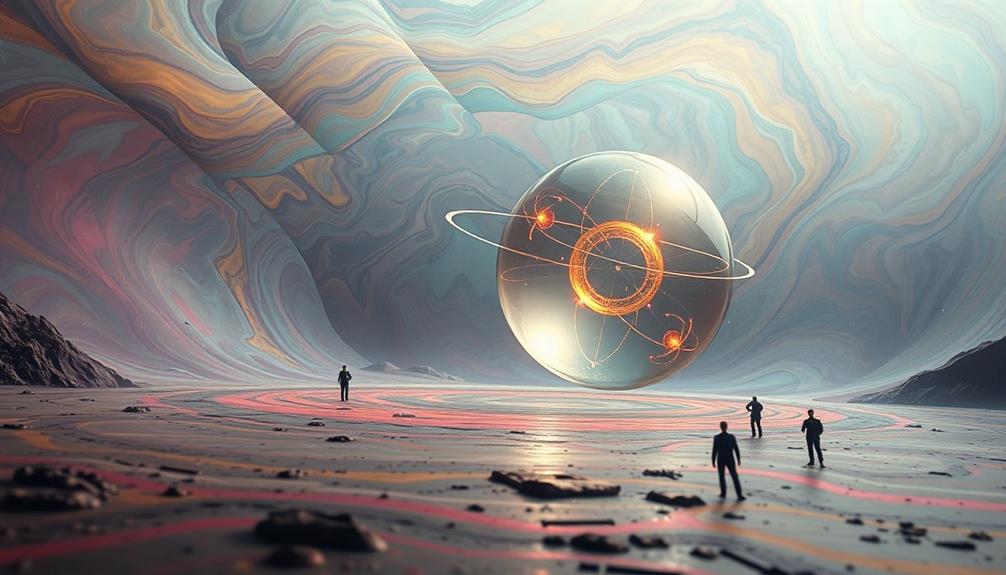
When you measure a quantum system, you actually change its state, leading to something called wave function collapse. This phenomenon underscores the complex relationship between observation and reality, much like the importance of early detection in managing public health.
You'll also encounter the Heisenberg uncertainty principle, which shows that some properties can't be known at the same time with perfect accuracy. Plus, observing a particle can drastically alter its behavior, making quantum measurement a fascinating yet puzzling aspect of physics.
Measurement Effects on States
Measurement in the domain of quantum physics profoundly alters the state of particles, showcasing a unique interplay between observer and observed. When you perform a measurement on a quantum system, you effectively collapse its wave function. This collapse forces the particle to take on a definite state from a range of possibilities. This phenomenon highlights the observer effect, where the act of observing changes the very behavior of the quantum system.
Curiously, much like how ethical hacking employs proactive measures to safeguard systems, understanding quantum measurement requires a proactive approach to grasp its complexities key components of cybersecurity.
The randomness inherent in quantum behavior means that you can't predict the outcome of a measurement with certainty. Each time you measure a quantum object, you're introducing an element of unpredictability, which is one of the most intriguing aspects of quantum mechanics.
Thought experiments, like Schrödinger's cat, illustrate how a system can exist in superposition—being in multiple states—until you observe it.
In essence, your measurements not only reveal information about a quantum system but also play a critical role in determining its state. This interaction emphasizes the profound connection between the observer and the observed, making quantum measurement a fascinating yet perplexing topic in physics.
Heisenberg Uncertainty Principle
The Heisenberg uncertainty principle discloses a fundamental truth about quantum measurement: you can't know both the exact position and momentum of a particle at the same time. This principle highlights a significant limit in quantum mechanics, emphasizing that the more accurately you measure one property, the less precise your measurement of the other becomes. It stems from the inherent wave-like behavior of quantum particles.
In a similar way, understanding the efficiency of systems, such as heat pump technology, also reveals limitations and balances that need to be considered for ideal performance.
Here are some important implications of the uncertainty principle:
- It underscores the probabilistic nature of quantum states.
- Measuring a particle's position disturbs its momentum, leading to measurement limitations.
- It plays a critical role in phenomena like quantum tunneling.
- The uncertainty principle is mathematically expressed as Δx * Δp ≥ ℏ/2, where Δx is position uncertainty, Δp is momentum uncertainty, and ℏ is the reduced Planck's constant.
Understanding this principle is essential for grasping the complexities of quantum mechanics. It not only challenges our classical notions of measurement but also reveals the strange behavior of subatomic particles.
Embracing this uncertainty can transform your perspective on the quantum world.
Observer Influence on Outcomes
Understanding how an observer influences outcomes in quantum mechanics adds another layer to the complexities highlighted by the Heisenberg uncertainty principle. When you measure a quantum system, you cause it to collapse from a superposition of states into one definitive state. This phenomenon exemplifies the observer effect, where the very act of measurement alters the state of the quantum system.
For instance, when you measure a particle's position, its velocity changes, showcasing that measurement isn't merely a passive observation but an active process that transforms the system. Remarkably, the implications of observer influence resonate with concepts in various fields, such as AI ethics, where the impact of decisions shapes outcomes considerably.
Thought experiments like Schrödinger's cat illustrate this paradox further. Until you observe the cat, it exists in a superposition of being both alive and dead. Only upon observation does it take on one of these states.
Physicists continue to debate whether your knowledge influences reality or if measurement alone triggers this collapse. This ongoing discussion highlights the profound implications of how observation shapes quantum outcomes, making you ponder the nature of reality itself.
Fundamentally, your role as an observer is essential to understanding and interpreting the strange behaviors of quantum systems.
The Mystery of Superposition
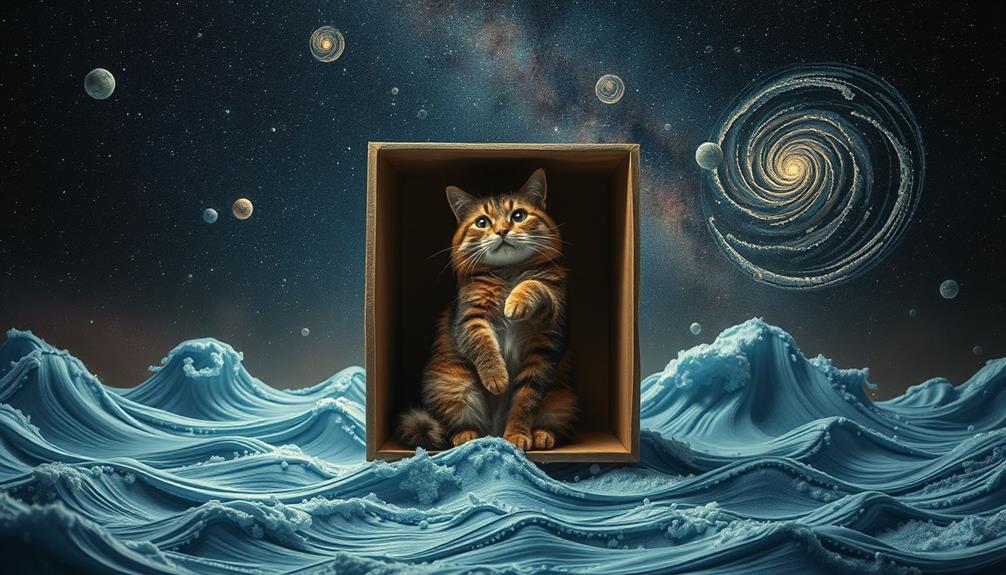
Exploring the mystery of superposition reveals a fascinating aspect of quantum mechanics where particles can exist in multiple states at once. This principle challenges your understanding of reality, as it implies that until you measure a particle, it doesn't settle into one specific state.
One classic example is Schrödinger's cat, which is both alive and dead until observed. This curious phenomenon prompts us to reconsider how we perceive existence and reality itself, highlighting the importance of benefits of curiosity in understanding complex concepts.
In the quantum world, superposition has profound implications:
- Qubits in Quantum Computing: Unlike classical bits, qubits can represent both 0 and 1 simultaneously, allowing for faster processing.
- Interference Patterns: In the double-slit experiment, particles act like waves, creating distinct patterns due to their ability to occupy multiple states.
- Fragility of Superpositions: They're sensitive to external interactions, leading to decoherence, which collapses the quantum state into a definite classical state.
- Counterintuitive Quantum Behaviors: Superposition defies classical logic, making it essential for understanding quantum mechanics.
Exploring Quantum Entanglement
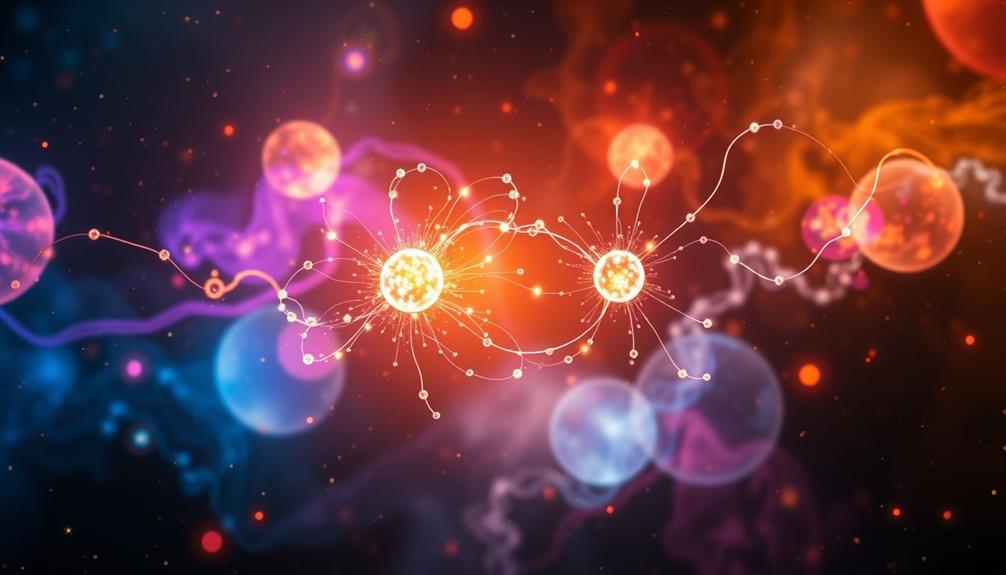
Quantum entanglement captivates with its mind-bending implications, where particles become intertwined in such a way that the state of one directly affects the other, no matter how far apart they are. This phenomenon, famously dubbed "spooky action at a distance" by Einstein, challenges our traditional understanding of communication and interaction.
Imagine two entangled particles; when you measure one, its state instantly determines the state of the other, regardless of the distance separating them. Recent advancements in technology have shown parallels with AI enhances accuracy in medical diagnoses, demonstrating how interconnected systems can lead to groundbreaking developments.
Recent experiments have pushed the boundaries of this concept, with scientists entangling six million photon pairs over 1,200 kilometers. Such astonishing feats showcase the power of quantum entanglement in securing communication, paving the way for advancements in quantum cryptography. By leveraging these particle correlations, we can achieve unparalleled security methods that traditional systems simply can't match.
Moreover, quantum entanglement is a cornerstone for the future of quantum computers. By enabling multiple qubits to work together simultaneously, it exponentially increases computational power.
As you explore this fascinating domain, you'll discover how entanglement not only reshapes our understanding of the universe but also revolutionizes technology and communication.
The Concept of Quantum Tunneling
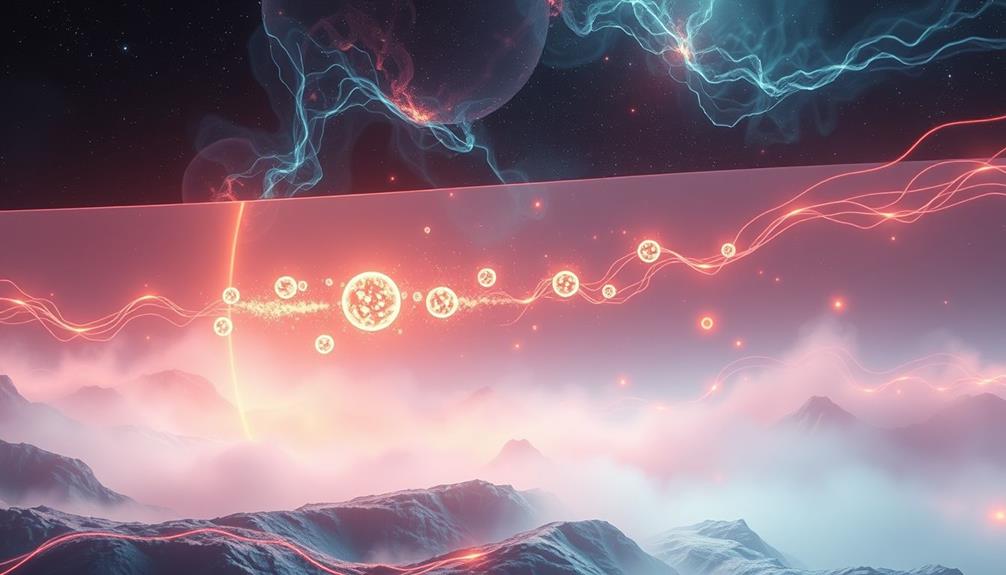
Quantum tunneling might sound complex, but it's simply a way for particles to pass through barriers they shouldn't be able to cross. This fascinating phenomenon plays a vital role in processes like nuclear fusion in stars and has practical applications in everyday technology, including touch screens.
Much like how solar panel efficiency relies on various factors for ideal performance, tunneling illustrates how particles can overcome seemingly insurmountable obstacles under the right conditions.
Let's break down how tunneling works and why it matters in both nature and our lives.
Tunneling Explained Simply
Understanding tunneling reveals a fascinating aspect of particle behavior that defies our classical intuition. In the subatomic world, particles don't just behave like tiny balls; they exhibit wave-like properties that allow them to exist in multiple places at once. This leads to a phenomenon known as quantum tunneling, where particles can pass through energy barriers they shouldn't be able to cross, according to classical physics.
This concept can be likened to how AI's impact on traditional music roles reshapes our understanding of creativity and production.
Here's how it works:
- Particles have a probability of being found on the other side of a barrier.
- Think of it like a marble that can "tunnel" through a hill instead of rolling over.
- Quantum tunneling is vital for processes like nuclear fusion in stars.
- It's also confirmed by experiments involving electrons in superconductors and alpha decay in radioactive materials.
This behavior is governed by a quantum rule called the uncertainty principle, which suggests that you can't know both the position and momentum of a particle precisely at the same time.
With quantum tunneling, particles challenge our understanding of movement and energy, pushing the boundaries of what's possible in the quantum domain.
Applications in Technology
The fascinating concept of tunneling has profound implications in modern technology, enabling innovations that harness the unique properties of particles. Quantum tunneling allows particles to pass through energy barriers that, according to classical physics, they shouldn't be able to cross. This phenomenon is essential for applications like tunnel diodes, which utilize quantum tunneling to facilitate current flow even in reverse bias, leading to high-speed electronic devices.
Additionally, quantum tunneling plays a significant role in scanning tunneling microscopes (STMs), enabling scientists to image surfaces at the atomic level by measuring tunneling currents between a sharp tip and a conducting surface.
You'll also find quantum tunneling in flash memory devices, where electrons tunnel through thin insulators to store data, providing faster and more efficient data storage solutions.
As research advances, quantum tunneling is set to revolutionize quantum computing. It allows qubits to shift between states seamlessly, paving the way for more complex computations and enhanced processing power.
These remarkable applications demonstrate how deeply interwoven quantum tunneling is in the technological innovations that shape our world today.
Historical Milestones in Quantum Theory
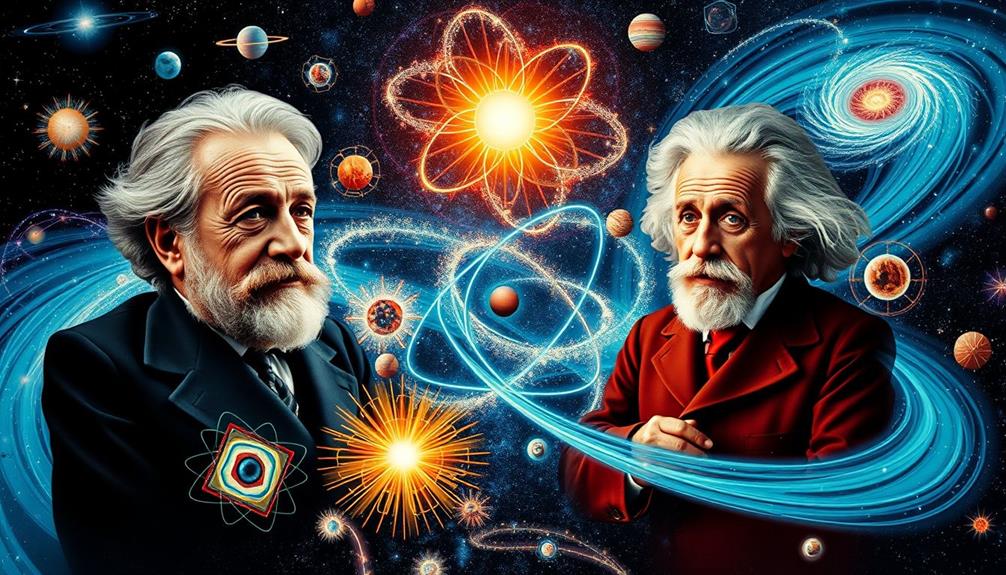
In the early 20th century, physicists began to unravel the mysteries of the microscopic world, leading to revolutionary ideas that transformed our understanding of nature. This period marked the birth of quantum theory, a framework that reshaped physics forever.
Key milestones in this journey include:
- Max Planck's quantization (1900): He introduced the idea of quantization to resolve issues with thermal radiation.
- Albert Einstein's photoelectric effect (1905): His work provided vital evidence for the particle nature of light, earning him the Nobel Prize in Physics in 1921.
- Niels Bohr's atomic model (1913): Bohr proposed quantized electron orbits, fundamentally altering our view of atomic structure.
- Werner Heisenberg's uncertainty principle (1927): This principle illustrated the limits of knowing both position and momentum simultaneously.
Additionally, Erwin Schrödinger's wave equation (1926) introduced wave functions, highlighting the probabilistic nature of quantum particles.
These milestones not only expanded scientific knowledge but also laid the groundwork for the modern interpretations of quantum mechanics, emphasizing that the universe operates in ways that often defy our everyday experiences.
Practical Applications of Quantum Physics
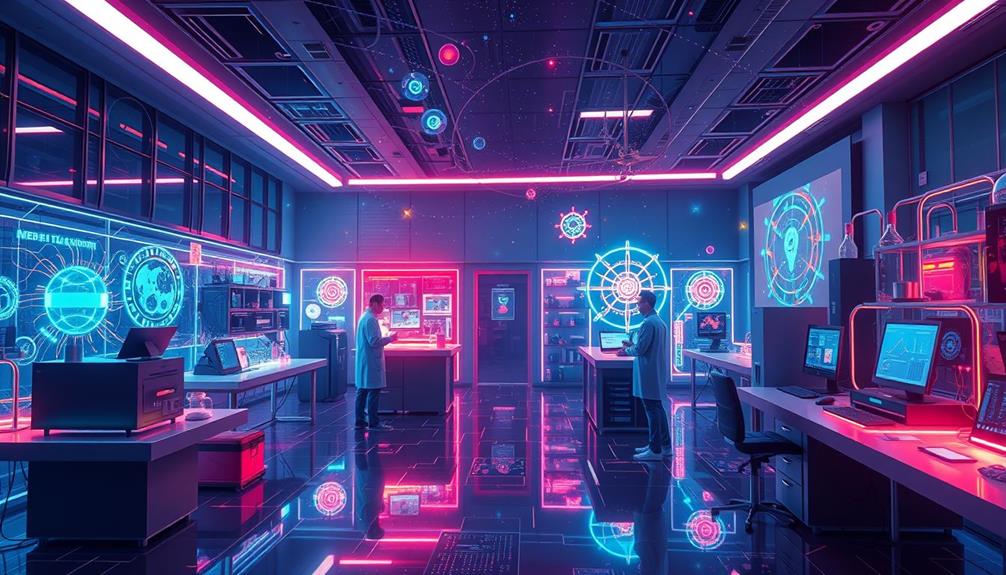
While many may associate quantum physics with abstract theories, its practical applications are woven into the fabric of everyday life.
Take lasers, for example; they're based on quantum mechanics and are essential in everything from barcode scanners to laser surgery. These technologies illustrate how quantum principles can transform daily activities.
Then there's quantum computing, which harnesses superposition and entanglement to perform calculations exponentially faster than classical computers. This capability could revolutionize fields like cryptography and drug discovery, making processes that once took years achievable in mere moments.
In the medical field, MRI scanners utilize quantum mechanics to produce detailed images of your body's internal structures, greatly enhancing diagnostics.
Furthermore, quantum cryptography offers secure communication channels that are theoretically immune to eavesdropping, ensuring your data remains safe.
Future Directions in Quantum Research
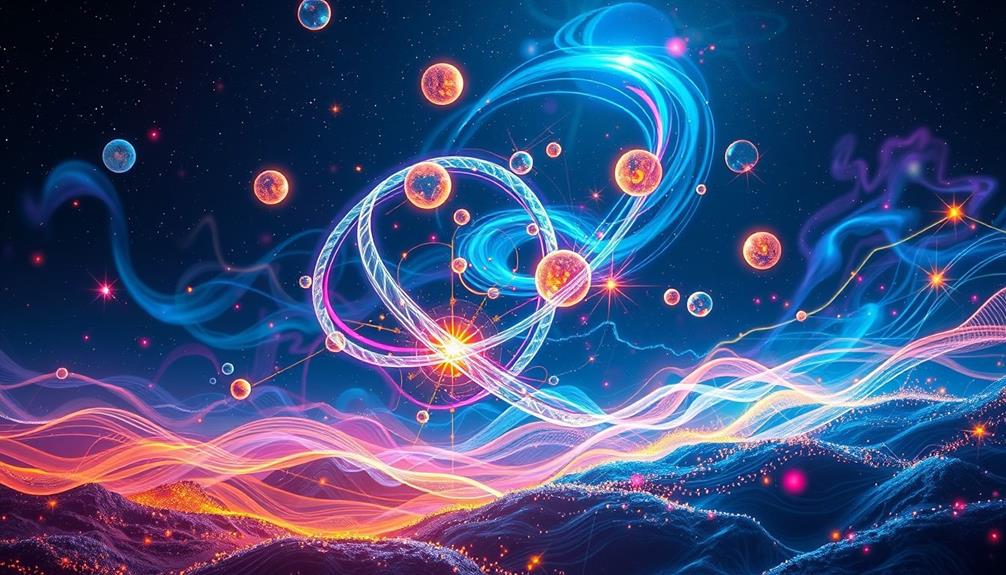
As you look ahead in quantum research, you'll find exciting developments in scalable quantum computers that promise to revolutionize processing power.
Researchers are also exploring quantum biology to uncover how quantum effects influence natural processes like photosynthesis.
Additionally, investigations into quantum gravity aim to bridge the gap between quantum mechanics and general relativity, potentially reshaping our understanding of the universe.
Scalable Quantum Computers
Looking ahead, scalable quantum computers promise to transform the landscape of computing by harnessing the unique properties of qubits. These quantum particles can exist in superposition, allowing for calculations that can be thousands of times faster than those performed by classical computers. Major tech companies like IBM and Google are leading the charge, with IBM even providing access to its quantum computers for research and experimentation.
To fully realize the potential of scalable quantum computers, researchers focus on several key areas:
- Developing stable, long-lived entangled qubits to prevent decoherence
- Increasing the number of qubits to enhance computational power
- Creating quantum algorithms like Shor's algorithm to revolutionize cryptography
- Exploring diverse applications, including drug discovery and complex modeling
As the field advances, you'll see breakthroughs in various scientific domains. The successful implementation of scalable quantum computers could redefine what's computationally possible, tackling optimization problems and enabling computations that are currently infeasible.
Embracing these innovations will pave the way for a new era in computing and open up exciting opportunities across numerous fields.
Quantum Biology Exploration
Exploring the intersection of quantum mechanics and biological processes reveals fascinating insights into how life operates at the molecular level.
Quantum biology investigates quantum phenomena, such as superposition and entanglement, to uncover mechanisms that enhance life's efficiency. For instance, research shows that quantum coherence plays an essential role in how birds navigate. Experiments suggest that entangled particles in their eyes might help them sense the Earth's magnetic field, greatly improving their migratory abilities.
Moreover, quantum tunneling could explain how enzymes catalyze reactions more efficiently. In this process, particles can pass through energy barriers, which speeds up reactions in biological systems. This could revolutionize our understanding of metabolic processes and enzyme function.
Ongoing investigations aim to bridge the gap between quantum mechanics and living organisms, offering insights that challenge traditional biology.
As you explore deeper into quantum biology, you'll discover how quantum entanglement and other phenomena might underpin the very fabric of life. This exciting field holds the potential to reshape our comprehension of life's fundamental processes, inviting us to rethink what we understand about nature's intricate workings.
Quantum Gravity Investigations
At the heart of modern physics, quantum gravity investigations aim to unify quantum mechanics with general relativity, offering an extensive framework for understanding gravity at the quantum level. Researchers are diving into various theoretical frameworks to describe how spacetime behaves in the quantum domain. Notable approaches include:
- String theory: Proposes that fundamental particles are one-dimensional "strings."
- Loop quantum gravity: Suggests that spacetime itself is quantized, composed of discrete units.
- Experimental projects: Initiatives like the LIGO observatory are actively searching for gravitational waves to provide insights into quantum gravity phenomena.
- Applications: Understanding quantum gravity could explain black hole dynamics and the conditions of the early universe.
As you explore these investigations, you'll see that quantum objects and their interactions challenge our classical notions of gravity.
The concept of spacetime quantization is particularly intriguing, hinting that our understanding of gravity may evolve dramatically. Grasping quantum gravity is essential for pushing the boundaries of theoretical physics, potentially leading to groundbreaking advancements in how we perceive the universe.
The journey into this complex landscape might just reveal secrets that redefine our fundamental comprehension of reality.
Challenges and Intrigues of Quantum Science
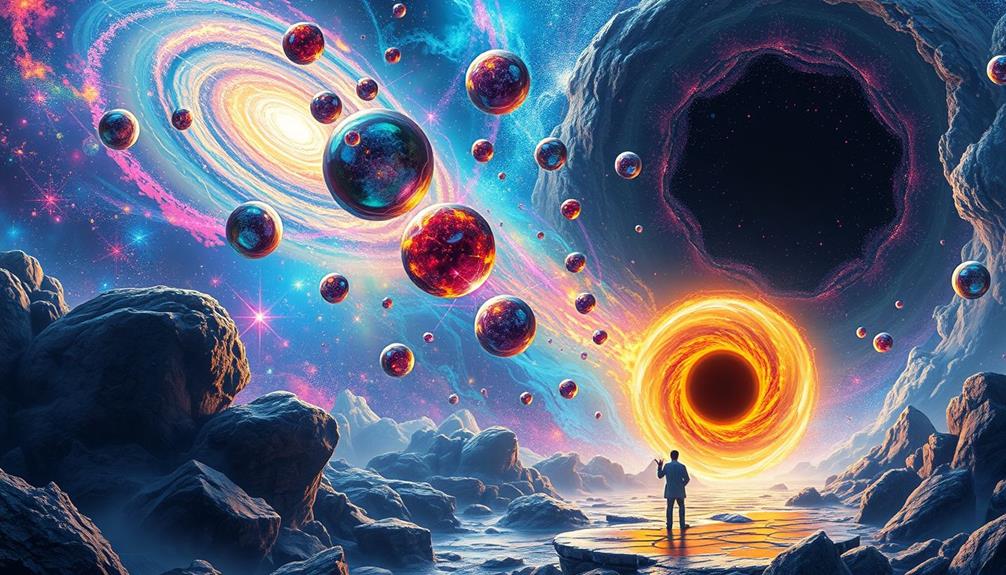
The challenges and intrigues of quantum science fascinate both researchers and enthusiasts alike. As you explore this intriguing domain, you'll discover key concepts that defy everyday logic. For example, superposition allows particles to exist in multiple states at once, but this phenomenon collapses into a definite state upon measurement. This brings us to the measurement problem, which questions how observation influences quantum events and highlights the perplexity of reality.
Another enthralling aspect is quantum entanglement, which creates instantaneous correlations between particles, regardless of distance. This phenomenon challenges classical understandings of locality and raises intriguing questions about information transfer in the universe.
Here's a concise overview of these challenges:
Concept | Description |
---|---|
Superposition | Particles exist in multiple states until measured. |
Measurement Problem | Observation can alter the outcome of quantum events. |
Quantum Entanglement | Instantaneous correlations between distant particles. |
Ongoing research aims to unify quantum mechanics with general relativity, aiming to bridge the gaps between the very small and the very large. Each breakthrough reveals new mysteries, ensuring quantum science remains an exciting field of exploration.
Conclusion
In the bizarre domain of quantum physics, you discover a world that defies your everyday logic. The idea that particles can exist in multiple states at once or be instantly connected across vast distances challenges everything you thought you knew. As researchers explore deeper, they uncover not just the mysteries of the universe but also potential technologies that could revolutionize our lives. So, are you ready to embrace the strange and unpredictable nature of reality?